Author affiliations: Department of Orthopaedic Surgery, Kobe University Graduate School of Medicine, Kobe, Japan.
About 5–10% of bone fractures fail to heal after conventional treatment. Stem cell therapy is promising for treating these cases, but initial results using mesenchymal stem cells have been disappointing. In a phase I/II clinical study, researchers associated with the Translational Research Center for Medical Innovation (TRI) in Japan have shown that transplantation of autologous CD34+ cells from peripheral blood in patients with non-union fractures is safe and effective way to heal such non-union fractures.
1. INTRODUCTION
Recent years have seen remarkable developments in the field of regenerative medicine as a result of research into embryonic stem cells and induced pluripotent stem cells. However, unresolved issues with the use of these so-called pluripotent cells, including ethical concerns and the potential for neoplastic cell transformation, make their clinical application problematic. Research into organogenesis and tissue regeneration has hence mainly focused on somatic stem cells. Regeneration of bone and cartilage using mesenchymal stem cells derived from the bone marrow or the synovial membrane is now attracting attention in orthopaedics and has already been used clinically. However, serious disadvantages remain, including the invasiveness of bone marrow collection, the difficulty and slowness of cell culture techniques, and the limited effectiveness of therapy. Thus, less invasive and more effective therapies are needed.
Vascular research has been conducted to establish the pathogenesis and improve the treatment of vascular diseases. Developments up until now in this field have not always been comparable with the most advanced research in fields such as oncology, hematology and neurology. However, the recent detailed characterization of the differentiation mechanisms involved in vascularization has opened up a new field of vascular research, incorporating trends in fields such as hematology and embryology. In the field of regenerative medicine, which is now part of mainstream medicine, research into organogenesis and tissue regeneration using various stem cells is underway, but many aspects of the mechanisms by which the vascular system can be reconstructed to nourish regenerated organs and tissues remain unclear. Vasculogenesis thus has the potential to play a key role in the development of regenerative medicine. After Asahara et al. discovered human peripheral blood vascular endothelial progenitor cells (EPCs) in 19971, several studies have elucidated the mechanism of vasculogenesis involving EPCs, in addition to that of conventional angiogenesis involving pre-existing vascular endothelial cells2,3. Revascularization is currently performed as therapy for lower-limb ischemia and ischemic cardiac diseases. The significance of vascularization, particularly for bone regeneration, has long been recognized in orthopaedics.
This article presents an overview of basic research, non-clinical studies and a phase I/II clinical study to investigate the in vivo kinetics of EPCs/peripheral blood CD34+ cells and bone revascularization therapy using EPCs/peripheral blood CD34+ cells, which have great potential for fracture treatment.
2. ANGIOGENESIS AND VASCULOGENESIS
Moses Judah Folkman first established the concept of angiogenic growth factor and its application to therapy, and he showed that angiogenic growth factors were expressed as a result of cancer growth and infiltration. He defined angiogenesis as the process of forming new blood vessels from pre-existing adjacent blood vessels by the proliferation and migration of vascular endothelial cells induced by growth factors4. The recent discovery of various growth factors, including vascular endothelial growth factor (VEGF), has led to the clinical application of therapy that promotes angiogenesis. However, some patients derived no clear benefit from this therapy. In these patients, the response of pre-existing vascular endothelial cells to vascular growth factors was probably lower at the ischemic lesion site. Therefore, the next challenge was to deliver highly responsive vascular endothelial cells to ischemic lesions.
In 1997, Asahara et al. reported that EPCs were present in adult blood circulation and involved in vascularization in severe ischemic lesions1. This mechanism was found to be consistent with vasculogenesis, a process previously thought to occur only during fetal life, in which undifferentiated vascular EPCs migrate to a local site, where they reside, proliferate and differentiate to form a new blood vessel. This discovery led to the development of a concept distinct from angiogenesis, which is the conventional concept of vascularization in adults, where vascular endothelial cells proliferate and migrate to adjacent pre-existing blood vessels. When ischemia, cancer, wound healing or neovascularization in the uterus and the ovary were induced in a transgenic mouse model of bone-marrow transplantation that expressed β-galactosidase following expression of vascular endothelial cell-specific genes (Flk-1, Tie-2), new blood vessels formed by cells expressing donor bone marrow–derived Flk-1 or Tie- 25,6. These studies showed that vascularization is achieved by both vasculogenesis and angiogenesis in adults as well as in fetal life. Neovascularization in adults is now thought to be mediated by an interaction between angiogenesis and vasculogenesis.
3. FRACTURE HEALING BY PERIPHERAL BLOOD EPCS/CD34+ CELLS
Bone fractures usually heal through anatomical reduction and rigid immobilization. However, bone union fails to occur (non-union fractures) in 5–10% of patients with fractures, partly because appropriate circulation is not achieved at the fracture site. Autologous free cancellous bone grafting is performed and may be followed by vascularized bone grafting with a focus on revascularization, but healing of a non-union fracture is often difficult. We therefore focused on neovascularization through cell therapy using EPCs and conducted basic experiments to examine the usefulness of this therapy for non-union fractures in preclinical animal studies.
In a preliminary study, we analysed the in vivo kinetics of EPCs in the fracture healing process by developing a mouse model of closed fracture and using fluorescence-activated cell sorting. EPCs were found to increase in the bone marrow and the peripheral blood after fracture. Subsequently, to demonstrate local differentiation of bone marrow–derived EPCs into vascular endothelial cells, double immunostaining of Sca1 and CD31 in a Tie2/ LacZ transgenic mouse model of bone marrow transplantation was performed. It showed that bone marrow–derived EPCs differentiated into vascular endothelial cells at the fracture site. These results demonstrated that fracture-induced mobilization of EPCs from the bone marrow to the peripheral blood occurs and that EPCs contributed to healing at the fracture site7. Other research groups have also reported that EPCs were mobilized to the fracture site in humans8 and to the distraction osteogenesis site in a rat distraction osteogenesis model9. These reports support our findings.
Given that revascularization using peripheral blood cells was possible, it was logical to assume that differentiation of human peripheral blood CD34+ cells into vascular endothelial cells would promote bone regeneration. In our next study, we therefore intravenously transplanted 105 CD34+ cells collected from the peripheral blood of healthy humans into a nude rat model of non-union fracture and demonstrated that these cells accumulated at the non-union site and contributed to fracture healing by inducing an environment suitable for healing through both vasculogenesis and osteogenesis (Fig. 1)10. Radiological and histological assessments showed that non-union was still present in all animals in the control groups (one control group injected with phosphate-buffered saline [PBS] and one transplanted with human mononuclear cells) 8 weeks postoperatively, whereas bone union was achieved in 66% of animals at 4 weeks and in all animals at 8 weeks in the CD34+ cell transplant group (Table 1A). Mechanical assessment by a three-point bending test demonstrated significantly stronger fracture healing in the CD34+ cell transplant group than in the control groups.
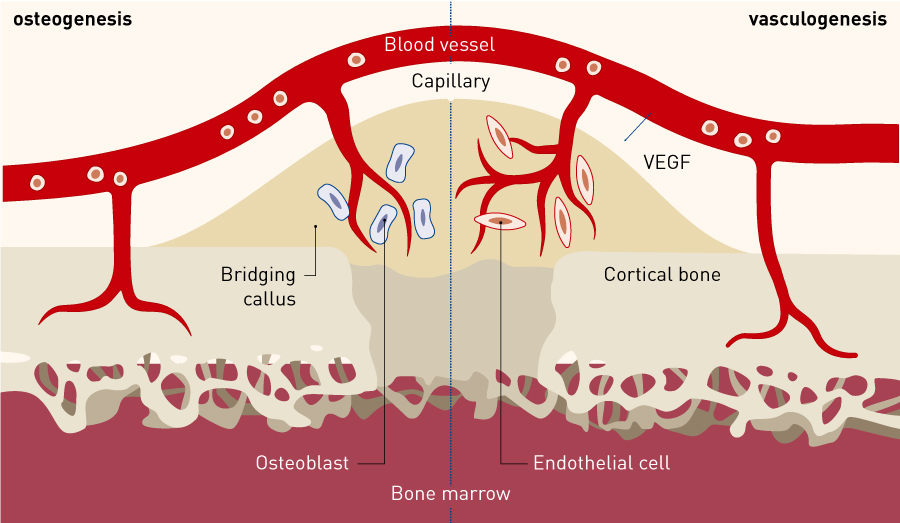
Figure 1. Kinetics of peripheral blood CD34+ cells in fracture healing. Intravenously transplanted human peripheral blood CD34+ cells were found to accumulate at the nonunion site, induce an environment suitable for fracture healing at the non-union site through both vasculogenesis and osteogenesis, and contribute to fracture healing.
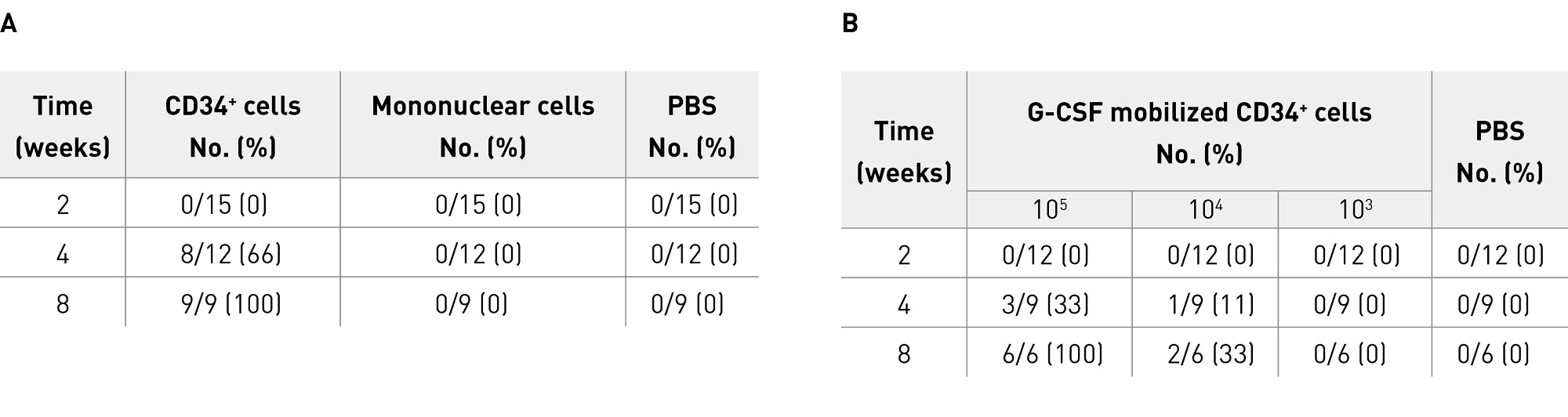
Table 1. Fracture healing following transplantation of human peripheral blood CD34+ cells into a nude rat model (basic nonclinical studies)
Fluorescently labeled CD34+ cells were found to accumulate preferentially at the non-union site and contribute to revascularization and bone regeneration in collaboration with rat-derived endothelial cells and osteoblasts. Two weeks after transplantation, histological and molecular biological analyses showed the presence of human-derived mature vascular endothelial cells and osteoblasts at the non-union site, demonstrating that CD34+ cells had differentiated into mature endothelial cells and osteoblasts. Single-cell reverse transcriptase polymerase chain reaction (RT-PCR) analysis showed that approximately 20% of CD34+ cells co-expressed osteocalcin, an osteoblast-specific marker, immediately after isolation, and that human CD34+ cells appeared to directly differentiate into osteoblasts without fusing with rat cells. Determination of the microvessel density and the osteoblast density in rats by immunostaining at the non-union site 2 weeks after transplantation showed that angiogenesis and osteogenesis were significantly increased in the CD34+ cell transplant group compared with both control groups. Because RT-PCR analysis identified angiogenic factors such as human-derived VEGF at the non-union site, the CD34+ cells were considered to play a paracrine role locally. Administration of soluble Flt1, a VEGF antagonist, inhibited not only angiogenesis but also osteogenesis, showing that angiogenesis by CD34+ cells plays a key role in the fracture healing process.
With eventual clinical application in mind, we also conducted an experiment to confirm that locally transplanted human peripheral blood CD34+ cells induced an environment suitable for fracture healing at the non-union site through both vasculogenesis and osteogenesis and contributed to fracture healing in a dose-dependent manner11. First, peripheral blood granulocyte colony stimulating factor (G-CSF)-mobilized CD34+ cells were transplanted locally to the non-union site of the same animal model (using atelocollagen as a carrier). The numbers of cells transplanted were based on the results of the above-described intravenous transplantation. Radiological and histological assessments showed that bone union was achieved in 33% of animals at 4 weeks and all animals at 8 weeks after transplantation of 105 cells, and in 11% of animals at 4 weeks and 33% at 8 weeks after transplantation of cells, but not after transplantation of 103 cells or in PBS controls (Table 1B). Determination of the microvessel density and the osteoblast density at the non-union site by immunostaining at 2 weeks showed dose-dependent increases in angiogenesis and osteogenesis. Greater levels of angiogenesis and osteogenesis were observed after transplantation of 104 cells and 105 cells than after transplantation of 103 cells or PBS injection. These results suggest that local transplantation of peripheral blood CD34+ cells would be useful as a new treatment option for non-union fractures.
To confirm the superiority of human peripheral blood CD34+ cells over mononuclear cells, we transplanted both cell types locally to the non-union site of the nude rat non-union fracture model and compared their effects. Transplantation of 107 mononuclear cells induced an environment suitable for fracture healing through both vasculogenesis and osteogenesis and contributed to fracture healing, but the efficacy was significantly inferior to that of transplantation of 105 CD34+ cells as measured by microvessel density, osteoblast density, and the density of human-derived mature vascular endothelial cells and osteoblasts, as well as by radiological, histological and mechanical assessments12. These effects of CD34+ and mononuclear cell transplantation have been confirmed not only in this refractory non-union fracture model but also in the established non-union model13. The results were attributed partly to infiltration of more inflammatory cells in the mononuclear cell transplant group than in the CD34+ cell transplant group.
Further characterization of the mechanisms involved in fracture healing associated with EPCs would lead to more improvement in therapeutic effects. We therefore investigated the healing effects of mobilization of EPCs to the non-union site by sustained released G-CSF14 and simvastatin15, the healing effect of extracellular mobilization of adaptor protein Lnk16,17 mediated by SCF/c-Kit signalling, and mobilization of EPCs mediated by SDF- 1/CXCR4 signalling18. All studies confirmed that EPCs are closely associated with fracture healing.
4. CLINICAL STUDY: BONE REVASCULARIZATION BY TRANSPLANTATION OF PERIPHERAL BLOOD CD34+ CELLS
Based on the above-described non-clinical research results, a phase I/II clinical trial of bone revascularization by transplantation of autologous peripheral blood CD34+ cells was conducted in patients with non-infectious, non-union fractures, following approval by the Review Committee on Clinical Research of Human Stem Cells on 4 September 2009. An advantage of bone revascularization therapy by transplantation of autologous peripheral blood CD34+ cells over conventional therapies is that it regenerates both bone and new blood vessels after proliferation of cells in an undifferentiated state and subsequent differentiation at the non-union site.
Eligible patients (two with femoral non-union fractures and five with tibial non-union fractures) aged between 20 and 70 years provided written informed consent in person. After obtaining consent, screening tests, a case review meeting and an eligibility assessment were conducted and patients were then enrolled. CD34+ cell isolation, non-union surgery and cell transplantation were performed at the Institute of Biomedical Research and Innovation, and patients were then transferred to Kobe University Hospital for observation.
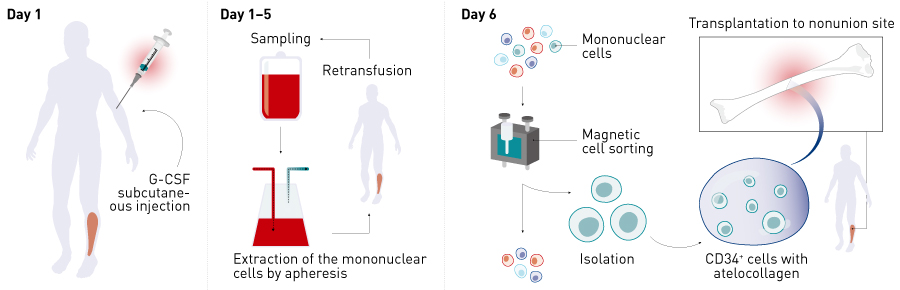
Figure 2. Overview of the clinical study. CD34+ cells were mobilized into the peripheral blood by subcutaneous injection of G-CSF, mononuclear cells were collected by apheresis,and then CD34+ cells were isolated by magnetic-activated cell sorting. Non-union surgery (improvement of internal fixation as necessary in addition to autologous bone grafting) was performed on day 6 and 5 ×105 cells kg–1 of autologous peripheral blood CD34+ cells were transplanted using atelocollagen as a carrier. G-CSF, granulocyte colony stimulating factor.
G-CSF was subcutaneously injected for 5 days to mobilize CD34+ cells into the peripheral blood. Mononuclear cells were collected by apheresis, and CD34+ cells were isolated by magnetic-activated cell sorting. Treatment involved non-union surgery (to improve internal fixation as needed in addition to autologous bone grafting) on day 6 and transplantation of 5 × 105 cells kg−1 of autologous peripheral blood CD34+ cells using atelocollagen as a carrier (Fig. 2). The primary endpoints were safety and the presence or absence of radiological fracture healing 12 weeks after surgery.
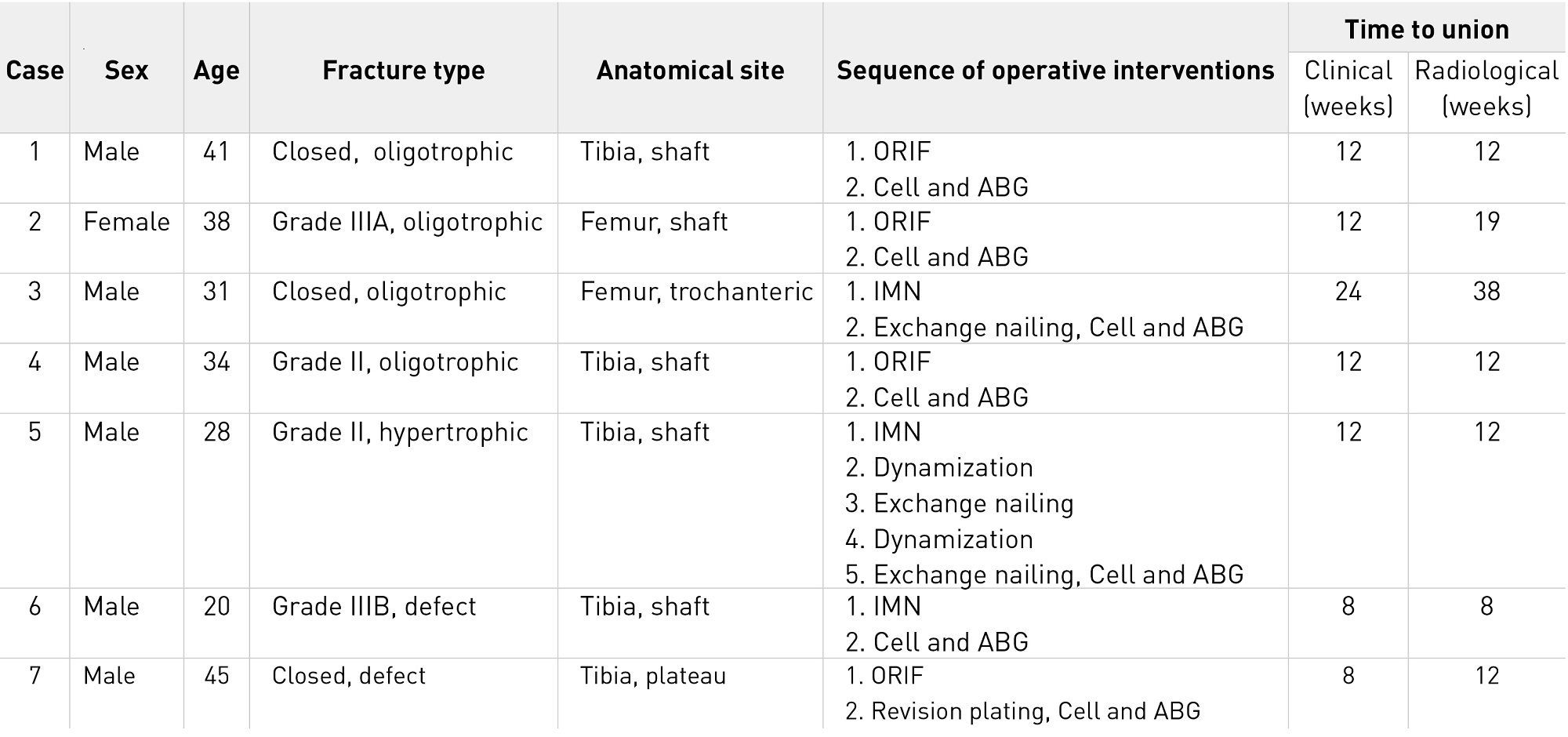
Table 2. Clinical and radiological outcomes for each patient in the phase I/II clinical study. Abbreviations: ABG, autologous bone grafting; Cell, transplantation of autologous peripheral blood CD34+ cells; IMN, intramedullary nailing; ORIF, open reduction and internal fixation.
We first reported safety and efficacy in a patient who had tibial non-union19. The mean postoperative time to clinical bone union was 12.6 weeks, and the mean postoperative time to radiological bone union was 16.1 weeks (Table 2)20. The efficacy of the therapy was demonstrated — 71.4% of patients achieved radiological bone union at 12 weeks after surgery, the primary endpoint, compared with a healing rate of 18.1% in 11 patients with femoral or tibial non-union who underwent concomitant bone grafting in the same medical institution, a patient group selected as a historical control (Table 3)20. The safety evaluation 1 year after surgery showed no serious adverse events.
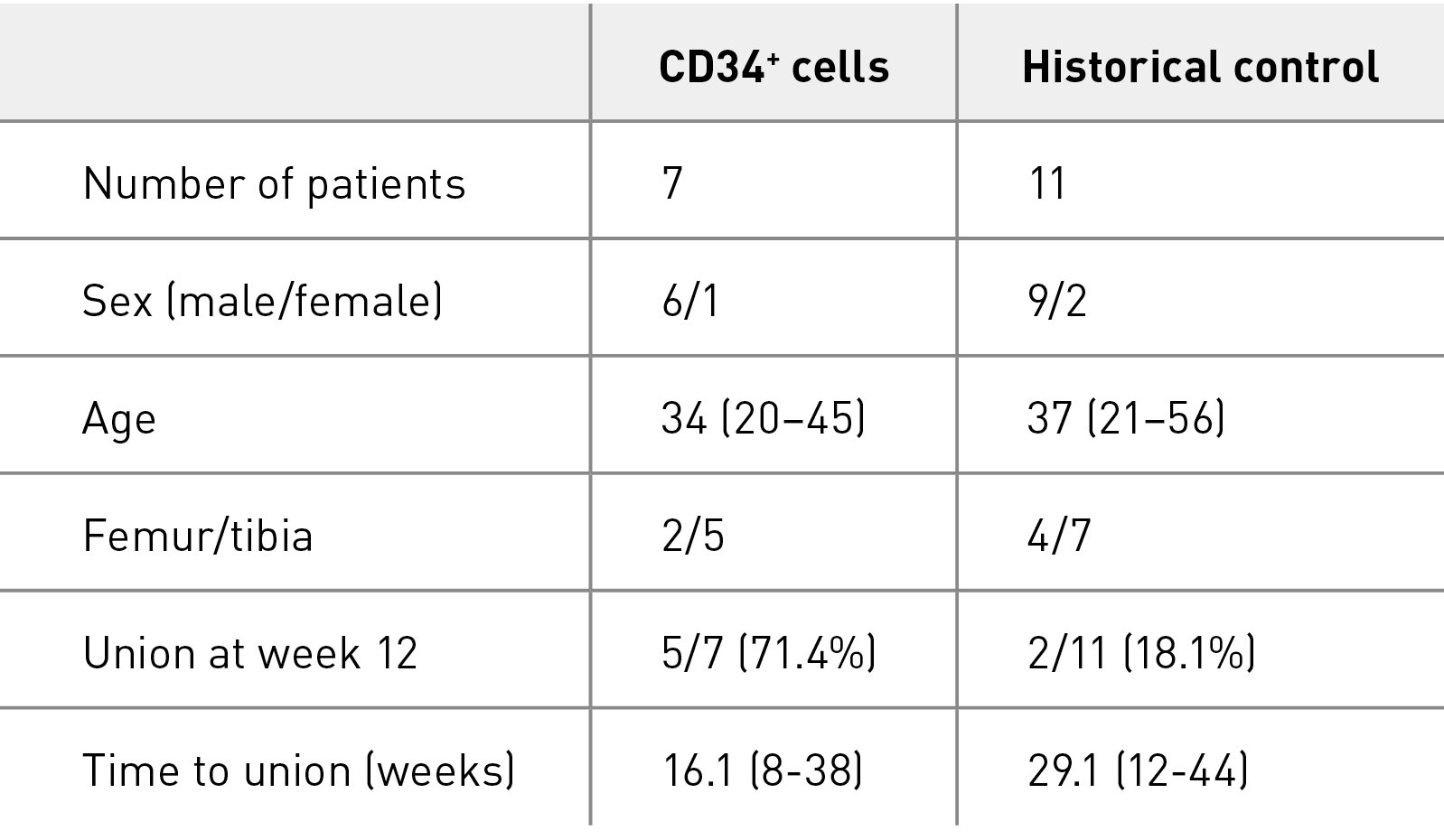
Table 3. Comparison of phase I/II study with historical control
5. FUTURE PROSPECTS
The significance and efficacy of EPCs/CD34+ cells in fracture healing described so far have attracted the attention of other research groups in recent years, and the results of basic research continue to accumulate. The effect of mobilization of EPCs to the peripheral blood has been reported for the rat model of tibial distraction osteogenesis21 and the mouse model of femoral fracture22 as well as for tibial long bone fracture in humans8,9. As well as in our immunodeficient rat non-union fracture model10–12, immunodeficient rat non-union model13, and rat femoral defect model23,24, the efficacy of EPCs/CD34+ cells in the fracture healing process has also been reported for the ovine tibial defect model25. Thus, the importance of EPCs/CD34+ cells has been increasingly recognized in recent years26–29.
The phase I/II clinical study demonstrated the efficacy and safety of this therapy in patients with non-union fractures. With the aim of establishing this therapy as a standard of care, we need to conduct a multicentre, investigator-initiated study in a larger number of patients. Transplantation of peripheral blood CD34+ cells, a novel therapy for non-union fractures, has already demonstrated high efficacy and safety in animal experiments and early clinical studies, and excellent therapeutic effects are expected in future extensive clinical applications.
References
- Asahara, T., Murohara, T., Sullivan, A., Silver, M., van der Zee, R. et al. Isolation of putative progenitor endothelial cells for angiogenesis. Science 275, 964–966 (1997). | article
- Kawamoto, A., Katayama, M., Handa, N., Kinoshita, M., Takano, H. et al. Intramuscular transplantation of G-CSF-mobilized CD34+ cells in patients with critical limb ischemia: a phase I/IIa, multicenter, single-blinded, dose-escalation clinical trial. Stem Cells 27, 2857–2864 (2009). | article
- Losordo, D. W., Schatz, R. A., White, C. J., Udelson, J. E., Veereshwarayya, V. et al. Intramyocardial transplantation of autologous CD34+ stem cells for intractable angina: a phase I/IIa double-blind, randomized controlled trial. Circ. 115, 3165–3172 (2007). | article
- Folkman, J. & Klagsbrun, M. Angiogenic factors. Science 235, 442–447 (1987). | article
- Asahara, T., Masuda, H., Takahashi, T., Kalka, C., Pastore, C. et al. Bone marrow origin of endothelial progenitor cells responsible for postnatal vasculogenesis in physiological and pathological neovascularization. Circ. Res. 85, 221–228 (1999). | article
- Takahashi, T., Kalka, C., Masuda, H., Chen, D. Silver, M. et al. Ischemia- and cytokine-induced mobilization of bone marrow-derived endothelial progenitor cells for neovascularization. Nature Med. 5, 434–438 (1999). | article
- Matsumoto, T., Mifune, Y., Kawamoto, A., Kuroda, R., Shoji, T. et al. Fracture induced mobilization and incorporation of bone marrow-derived endothelial progenitor cells for bone healing. J. Cell. Physiol. 215, 234–242 (2008). | article
- Laing, A. J., Dillon, J. P., Condon, E. T., Street, J. T., Wang, J. H. et al. Mobilization of endothelial precursor cells: Systemic vascular response to musculoskeletal trauma. J. Orthop. Res. 25, 44–50 (2007). | article
- Lee, D. Y., Cho, T.-J. Lee, H. R., Park, M. S., Yoo, W. J. et al. Distraction osteogenesis induces endothelial progenitor cell mobilization without inflammatory response in man. Bone 46, 673–679 (2010). | article
- Matsumoto, T., Kawamoto, A., Kuroda, R., Ishikawa, M., Mifune, Y. et al. Therapeutic potential of vasculogenesis and osteogenesis promoted by peripheral blood CD34-positive cells for functional bone healing. Am. J. Pathol. 169, 1440–1457 (2006). | article
- Mifune, Y., Matsumoto, T., Kawamoto, A., Kuroda, R., Shoji, T. et al. Local delivery of granulocyte colony stimulating factor-mobilized CD34-positive progenitor cells using bioscaffold for modality of unhealing bone fracture. Stem Cells 26, 1395–1405 (2008). | article
- Fukui, T., Matsumoto, T., Mifune, Y., Shoji, T., Kuroda, T. et al. Local transplantation of granulocyte colony-stimulating factor-mobilized human peripheral blood mononuclear cells for unhealing bone fractures. Cell Transplant. 21, 707–721 (2012). | article
- Fukui, T., Mifune, Y., Matsumoto, T., Shoji, T., Kawakami, Y. et al. Superior potential of CD34-positive cells compared to total mononuclear cells for healing of nonunion following bone fracture. Cell Transplant. 24, 1379–1393 (2015). | article
- Ishida, K., Matsumoto, T., Sasaki, K., Mifune, Y., Tei, K. et al. Bone regeneration properties of granulocyte colony-stimulating factor via neovascularization and osteogenesis. Tissue Eng. 16, 3271–3284 (2010). | article
- Fukui, T., Ii, M., Shoji, T., Matsumoto, T., Mifune, Y. et al. Therapeutic effect of local administration of low-dose simvastatin-conjugated gelatin hydrogel for fracture healing. J. Bone Min. Res. 27, 1118–1131 (2012). | article
- Matsumoto, T., Ii, M., Nishimura, H., Shoji, T., Mifune, Y. et al. Lnk-dependent axis of SCF-cKit signal for osteogenesis in bone fracture healing. J. Exp. Med. 207, 2207–2223 (2010). | article
- Kawakami, Y., Ii, M., Matsumoto, T., Kawamoto, A., Kuroda, R. et al. A small interfering RNA targeting Lnk accelerates bone fracture healing with early neovascularization. Lab. Invest. 93, 1036–1053 (2013). | article
- Kawakami, Y., Ii, M., Matsumoto, T., Kuroda, R., Kuroda, T. et al. SDF-1/CXCR4 axis in Tie2-lineage cells including endothelial progenitor cells contributes to bone fracture healing. J. Bone Miner. Res. 30, 95–105 (2015). | article
- Kuroda, R., Matsumoto, T., Miwa, M., Kawamoto, A., Mifune, Y. et al. Local transplantation of G-CSF-mobilized CD34+ cells in a patient with tibial nonunion: a case report. Cell Transplant. 20, 1491–1496 (2011). | article
- Kuroda, R., Matsumoto, T., Niikura, T., Kawakami, Y., Fukui, T. et al. Local transplantation of granulocyte colony stimulating factor-mobilized CD34+ cells for patients with femoral and tibial nonunion: Pilot clinical trial. Stem Cells Transl. Med. 3, 128–134 (2014). | article
- Cetrulo, C. L., Knox, K. R., Brown, D. J., Ashinoff, R. L., Dobryansky, M. et al. Stem cells and distraction osteogenesis: Endothelial progenitor cells home to the ischemic generate in activation and consolidation. Plast. Reconstr. Surg. 116, 1053–1064 (2005). | article
- Laing, A. J., Dillon, J. P., Condon, E. T., Coffey, J. C., Street, J. T. et al. A systemic provascular response in bone marrow to musculoskeletal trauma in mice. J. Bone Joint Surg. 89B, 116–120 (2007). | article
- Atesok, K., Li, R., Stewart, D. J. & Schemitsch, E. H. Endothelial progenitor cells promote fracture healing in a segmental bone defect model. J. Orthop. Res. 28, 1007–1014 (2010). | article
- Ma, X.-L., Sun, X.-L., Wan, C.-Y., Ma, J.-X. & Tian, P. Significance of circulating endothelial progenitor cells in patients with fracture healing process. J. Orthop. Res. 30, 1860–1866 (2012). | article
- Rozen, N., Bick, T., Bajayo, A., Shamian, B., Schrift-Tzadok, M. et al. Transplanted blood-derived endothelial progenitor cells (EPC) enhance bridging of sheep tibia critical size defects. Bone 45, 918–924 (2009). | article
- Matsumoto, T., Kuroda, R., Mifune, Y., Kawamoto, A., Shoji, T. et al. Circulating endothelial/skeletal progenitor cells for bone regeneration and healing. Bone 43, 434–439 (2008). | article
- Atesok, K., Matsumoto, T., Karlsson, J., Asahara, T., Atala, A. et al. An emerging cell-based strategy in orthopaedics: endothelial progenitor cells. Knee Surg., Sports Traumatol., Arthrosc. 20, 1366–1377 (2012). | article
- Fadini, G. P., Rattazzi, M., Matsumoto, T., Asahara, T. & Khosla, S. Emerging role of circulating calcifying cells in the bone-vascular axis. Circ. 125, 2772–2781 (2012). | article
- Kuroda, R., Matsumoto, T., Kawakami, Y., Fukui, T., Mifune, Y. et al. Clinical impact of circulating CD34-positive cells on bone regeneration and healing. Tissue Eng. B Rev. 20, 190–199 (2014). | article